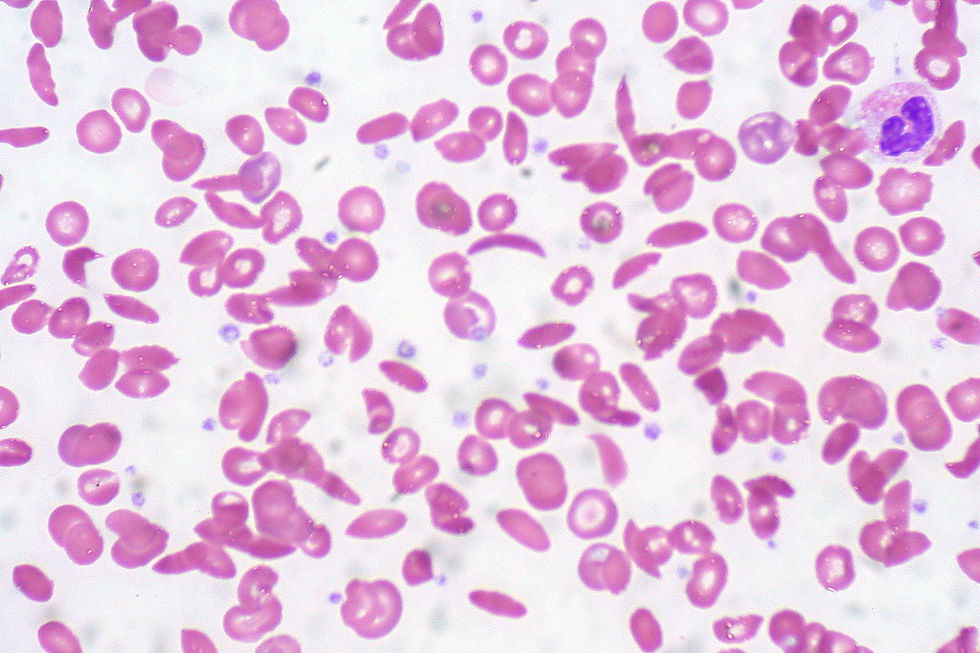
Abstract
Sickle cell disease is an autosomal recessive disorder characterized by C-shaped red blood cells. About 300,000 to 400,000 infants are born with this condition each year. 1 Blood and bone marrow stem cell transplantation are the only cure for this disease; however, they are associated with serious complications such as immunological mismatch that leads to host rejection. Moreover, frequent blood transfusion may cause iron overload, so additional therapy is required to remove the excess amount of iron from the patient. Interestingly, heterozygotes for sickle gene had been found to protect against the risk of dying from malaria, which might explain the high prevalence of sickle cell gene in Africa as it is a continent with highest incidence of malaria.2 On the other hand, homozygous for sickle gene leads to anemia, and in worst case, the sickling cells can clog the blood flow, resulting in acute chest syndrome and stroke. The CRISPR-Cas9 gene therapy has been developed to provide a lifelong treatment for sickle cell disease. Two therapeutic approaches can be accomplished by this technology: (1) The mutated HBB gene can be directly repaired, resulting in normal form of adult hemoglobin, and (2) Indirectly induce the expression of fetal hemoglobin (HbF) to replace the mutated adult hemoglobin. Several studies have been conducted to determine the efficacy of each approach, however, none compare the results between these two approaches. Therefore, the purpose of this research proposal is to investigate which of these two strategies yield better efficiency in restoring hemoglobin levels in-vitro model
Five keywords to find information about my topic: (1) HBB mutation, (2) Fetal hemoglobin (HbF) induction, (3) BLC11A enhancer, (4) CRISPR/Cas9 system, (5) homology-directed repair (HDR).
Research question:
Compare the efficacy of two sickle cell disease treatments using CRISPR to: (1) Induce fetal hemoglobin expression, (2) Correct HBB mutation to restore adult hemoglobin.
Hypothesis:
Using CRISPR to correct HBB mutation will be more effective, in terms of producing more hemoglobin, than using CRISPR to induce fetal hemoglobin.
Two specific aims that describe the goals:
To determine which CRISPR therapeutic strategies will lead to a higher hemoglobin level in treatment of sickle cell patients. To confirm that the knockout of BCL11A will lead to increase in expression of γ-globin, indicating production of HbF. To confirm that the knock-in of normal donor template will subsequently restore the function of adult hemoglobin.
Introduction:
Sickle cell disease is caused by a point mutation (substitution of A to T) in the sixth codon of human β-globin gene (HBB). 3 Particularly, the mutation occurs in the HBB allele of hemoglobin S (HbS), resulting in stiff and sticky red blood cell structure that can clump together and narrow the blood vessels. One of CRISPR-Cas9 mediated approaches is to delete the mutated sequence and knock in a normal template, which will restore the function of β subunit of adult hemoglobin. This will allow the normal form of hemoglobin to be produced. Another option is to use CRISPR-Cas9 mediated deletion in BLC11A enhancer. Beginning shortly before birth, BCL11A is responsible for suppressing the expression of fetal hemoglobin, allowing the adult hemoglobin to be expressed instead. Disruption of BCL11A sequence will lead to the production of γ-globin chains which in turn, combine with alpha globin chains and result in fetal hemoglobin (HbF). HbF can be used to replace the mutated adult hemoglobin.
Methods:
1. Brief summary of experiment (what, why, controls)
HEK293T was the chosen cell line for the transfection of plasmid spCas9 (BB)-2A-GFP. Different sgRNAs were required for HBB gene and BCL11A gene, because sgRNAs would guide Cas9 protein to cut at a specific location. The plasmid spCas9 (BB)-2A-GFP was digested, so that sgRNA could be inserted. The negative control was the uncut plasmid. In the HBB mutation sample, a donor template was introduced to allow the production of normal functional adult hemoglobin. In the BCL11A disruption sample, qPCR-RT was performed to evaluate the expression of BCL11A, subsequently confirming whether the disruption in BCL11A enhancer would lead to γ-globin expression. Lastly, to measure the expression of hemoglobin, the number of fluorescent cells were counted and compared between the samples.
2. General experimental setup
The information about genomic sequence of HBB gene was extracted from NCBI gene database. By using an online CRISPR design tool, several single-guided RNA (sgRNA) sequences were designed. One sgRNA was designed to specifically target BCL11A enhancer at +58 DNase I hypersensitive site (DHS) region, and one sgRNA was designed to specifically target exon 1 of HBB gene. 4,5 To produce short oligonucleotide fragments, synthetic complementary oligonucleotides which encode the gene-specific sgRNA was annealed into corresponding designed sgRNAs. These double-strand oligonucleotides were cloned into BsmBI-digested plasmid spCas9 (BB)-2A-GFP. The uncut spCas9 (Bb)-2A-GFP was used as a negative control. The three petri dishes containing HEK293T cell lines were obtained for 3 samples, labelled as: negative control (uncut plasmid), HBB mutation (HEK293T cells were transfected with plasmid DNA containing sgRNA that target HBB gene locus), and BCL11A disruption (HEK293T cells were transfected with plasmid DNA containing sgRNA that target BCL11A enhancer sequence).
2a. HBB mutation sample
In the HBB mutation labelled plate, a donor template which contains a normal sequence of HBB was introduced. As sgRNA directed Cas9 protein to bind and disrupt the HBB gene, the site of break could be used to knock-in a normal template sequence. Through homology-directed repair (HDR), the cells would repair the DNA break with the template, resulting in HBB expression.6 To evaluate HBB protein expression in the HBB mutation sample, western blotting was conducted. After 24 hours of post-transfection, the cells were stained with fluorescent and assessed under fluorescent microscope. The number of fluorescent and non-fluorescent cells were counted and recorded. Fluorescent cells corresponded to the cells that produced adult hemoglobin protein, and non-fluorescent cells were the ones that didn’t express adult hemoglobin production.
2b. BCL11A disruption sample
Quantitative RT-PCR was performed to evaluate the BCL11A gene expression level. The same technique was also used to determine whether the disruption stimulated γ-globin expression level. Western blot analysis was conducted to assess the fetal hemoglobin levels with highest γ-globin expression. After 24 hours of posttransfection, the cells were stained with fluorescent, and fluorescence microscopy was performed. The number of fluorescent and non-fluorescent cells were counted and recorded. Fluorescent cells represented the cells that produced fetal hemoglobin, and non-fluorescent cells were the ones that didn’t express fetal hemoglobin.
3. Samples comparison and predicted result
The number of fluorescent cells were compared between the negative control, HBB mutation, and BCL11A disruption plates. It could be possible that the induction of HbF was contributed by other factors as well, so the disruption of BCL11A enhancer alone would not lead to a high expression of HbF. The direct repair of HBB mutation was more likely to yield a higher number of hemoglobin expressions. Therefore, it was predicted that a higher number of fluorescent cells would be observed in HBB mutation plate, indicating more expression of hemoglobin. The negative control was also stained with fluorescent, and no fluorescent cells should be observed. Confounding factors could be the change in pH of the HEK293T cell lines that affect the fluorescence. Increased pH would result in more luminescence, leading to falsely determination that the cells expressed hemoglobin even though it didn’t.
Impact statement:
Gene therapy is well-known for its high efficacy; however, it always goes along with an extremely high cost with the average price of about 1 million dollars. The gene therapy for sickle cell disease isn’t an exception. The predicted to range from 1 to 3 million dollars. This unaffordable price prevents the accessibility of patients to the treatment, leading to the preference of going back to the traditional therapy (stem cell transplantation and blood transfusion). As a result, the pharmaceutical company won’t get enough profits to cover the investment cost, and eventually, the treatment will be withdrawn from the market. By determining which CRISPR-Cas9 approach in SCD treatment is more effective, researchers can focus on developing that treatment with a higher success chance. This can also allow the pharmaceutical companies to avoid the unnecessary investment on both treatments when knowing one of them doesn’t provide as much efficiency as the other one. Sickle cell disease currently has no permanent cure, this increases the need for developing a lifelong therapy for this condition. As this is an inherited disorder, gene therapy is the best option to provide a long-term treatment. Furthermore, stem cell transplantation is based on availability of donors, so patients have to wait for a very long time until a donor with closely matched bone marrow is found. Through the development of effective CRISPR/Cas9 gene editing therapy the need for stem cell donors as well as complications associated with stem cell transplantation will essentially be eliminated.
Annotated bibliography:
1. Kato GJ, Piel FB, Reid CD, et al. Sickle cell disease. Nature Reviews Disease Primers. 2018;4(1). doi: https://doi.org/10.1038/nrdp.2018.10
2. Luzzatto L. SICKLE CELL ANAEMIA AND MALARIA. Mediterranean Journal of Hematology and Infectious Diseases. 2012;4(1):e2012065-e2012065. doi: https://doi.org/10.4084/mjhid.2012.065
3. Batuhan Mert Kalkan, Ezgi Yağmur Kala, Melek Yüce, Medine Karadag Alpaslan, Fatih Kocabaş. Development of gene editing strategies for human β-globin (HBB) gene mutations. Gene. 2020;734:144398-144398. doi: https://doi.org/10.1016/j.gene.2020.144398
This article describes the method of how to target HBB gene by using CRISPR/Cas9 genome editing to introduce nucleotide alterations for correction of point mutations in the HBB gene that causes sickle cell disease in human cell line. Furthermore, the authors propose that co-delivery of donor DNA template leading to homology directed repair (HDR) mechanism, which subsequently, fixing the point mutations in HBB gene. The authors aim to develop a sickle cell disease specific gRNA with high affinity to HBB locus that is similar to the SCD mutation. This will allow repairment of HBB gene with different lengths of HDR templates to cover all possible mutations. Moreover, the article provides a detailed description of the location of HBB mutation in SCD, and this information was used as part of the introduction of my research proposal.
4. Khosravi M, Abbasalipour M, Jean-Paul Concordet, et al. Targeted deletion of BCL11A gene by CRISPR-Cas9 system for fetal hemoglobin reactivation: A promising approach for gene therapy of beta thalassemia disease. European Journal of Pharmacology. 2019;854:398-405. doi: https://doi.org/10.1016/j.ejphar.2019.04.042
This article describes how the use of CRISPR/Cas9 to delete 200bp of BCL11A erythroid enhancer will induce strong expression of γ-hemoglobin in K562 cell lines. The authors also contend that KU-812 and KG-1 cell lines are not good models for representing HbF reactivation through suppression of BCL11A. Additionally, the results from the study indicate that CRISP-Cas9 can also be used as a genome editing tool for treating β-thalassemia. Moreover, the authors design a specific gRNA to target BCL11A locus, and this information was used as part of the “general experimental setup” section to particularly inform the exact location of BCL11A gene that gRNA will aim at.
5. Cai L, Bai H, Mahairaki V, et al. A Universal Approach to Correct Various HBB Gene Mutations in Human Stem Cells for Gene Therapy of Beta-Thalassemia and Sickle Cell Disease. Stem Cells Translational Medicine. 2017;7(1):87-97. doi: https://doi.org/10.1002/sctm.17-0066
This article discusses how targeting different HBB mutations is still difficult as guide RNA and donor DNA template of each type of HBB mutation have to be identified and validated. The authors devise a strategy, aiming to achieve targeted insertion of HBB cDNA in exon 1 of HBB gene using Cas9 and two guide RNAs. Additionally, the authors also mentioned the drawbacks of the current gene therapy that use lentiviral vector to insert HBB coding sequences into patients as well as the disadvantage of bone marrow transplantation using hematopoietic stem cells (HSCs) from a donor with wildtype HBB gene. Moreover, the authors design a specific gRNA to target HBB locus, and this information was used as part of the “general experimental setup” section to particularly inform the exact location of HBB gene that gRNA will aim at.
6. Synthego | Full Stack Genome Engineering. Synthego.com. Published 2023. Accessed November 19, 2023. https://www.synthego.com/crispr-sickle-celldisease#:~:text=Using%20CRISPR%20to%20promote%20fetal,replacing%20the%20mutated%20adult%20hemoglobin.
The website provides definition of sickle cell anemia, the cause of SCD, inheritance pattern of SCD, the difference between SCD and β-thalassemia, and the two approaches of using CRISPR to treat SCD. The authors provide a brief explanation of how using CRISPR to repair mutation in adult hemoglobin gene can result in normal production of adult hemoglobin. In particular, CRISPR breaks DNA strand, and the donor template will be knocked in. Through homology-directed repair, the cell repairs the DNA break with the template, and normal hemoglobin will be produced. This summary of how using CRISPR to restore adult hemoglobin is straightforward and easy to understand, so it was used as part of the “HBB mutation sample” section to help the audience understand better.
Comentarios